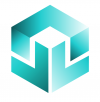
Levent DEMİRALP
Terms of Use
Website disclaimer
The information contained in this website is for general information purposes only. The information is provided by Precision Sealing Technology Limited and while we endeavour to keep the information up to date and correct, we make no representations or warranties of any kind, express or implied, about the completeness, accuracy, reliability, suitability or availability with respect to the website or the information, products, services, or related graphics contained on the website for any purpose. Any reliance you place on such information is therefore strictly at your own risk.
In no event will we be liable for any loss or damage including without limitation, indirect or consequential loss or damage, or any loss or damage whatsoever arising from loss of data or profits arising out of, or in connection with, the use of this website.
Through this website you are able to link to other websites which are not under the control of Precision Sealing Technology Limited. We have no control over the nature, content and availability of those sites. The inclusion of any links does not necessarily imply a recommendation or endorse the views expressed within them.
Every effort is made to keep the website up and running smoothly. However, Precision Sealing Technology Limited takes no responsibility for, and will not be liable for, the website being temporarily unavailable due to technical issues beyond our control.
This website and its content is copyright of Precision Sealing Technology Limited 2016. All rights reserved.
Any redistribution or reproduction of part or all of the contents in any form is prohibited other than the following:
you may print or download to a local hard disk extracts for your personal and non-commercial use only
you may copy the content to individual third parties for their personal use, but only if you acknowledge the website as the source of the material
You may not, except with our express written permission, distribute or commercially exploit the content. Nor may you transmit it or store it in any other website or other form of electronic retrieval system.
Tolerance Table
D | H6 | H7 | H8 | H9 | H10 | H11 | H12 | e9 | f7 | f8 | h8 | h9 | h10 | h11 |
0 < D ≤ 3 | +0,006 -0 | +0,01 -0 | +0,014 -0 | +0,025 -0 | +0,04 -0 | +0,06 -0 | -0,014 -0,039 | -0,006 -0,016 | -0,006 -0,02 | -0,006 -0,031 | +0 -0,014 | +0 -0,025 | +0 -0,04 | +0 -0,06 |
3 < D ≤ 6 | +0,008 -0 | +0,012 -0 | +0,018 -0 | +0,03 -0 | +0,048 -0 | +0,075 -0 | -0,02 -0,05 | -0,01 -0,022 | -0,01 -0,028 | -0,01 -0,04 | +0 -0,018 | +0 -0,03 | +0 -0,048 | +0 -0,075 |
6 < D ≤ 10 | +0,009 -0 | +0,015 -0 | +0,022 -0 | +0,036 -0 | +0,058 -0 | +0,09 -0 | -0,025 -0,061 | -0,013 -0,028 | -0,013 -0,035 | -0,013 -0,049 | +0 -0,022 | +0 -0,036 | +0 -0,058 | +0 -0,09 |
10 < D ≤ 18 | +0,011 -0 | +0,018 -0 | +0,027 -0 | +0,043 -0 | +0,07 -0 | +0,11 -0 | -0,032 -0,075 | -0,016 -0,034 | -0,016 -0,043 | -0,016 -0,059 | +0 -0,027 | +0 -0,043 | +0 -0,07 | +0 -0,11 |
18 < D ≤ 30 | +0,013 +0 | +0,021 +0 | +0,033 +0 | +0,052 +0 | +0,084 +0 | +0,13 +0 | -0,04 -0,092 | -0,02 -0,041 | -0,02 -0,053 | -0,02 -0,072 | +0 -0,033 | +0 -0,052 | +0 -0,084 | +0 -0,13 |
30 < D ≤ 50 | -0,016 +0 | -0,025 +0 | -0,039 +0 | -0,062 +0 | -0,1 +0 | -0,16 +0 | -0,05 -0,112 | -0,025 -0,05 | -0,025 -0,064 | -0,025 -0,087 | +0 -0,039 | +0 -0,062 | +0 -0,1 | +0 -0,16 |
50 < D ≤ 80 | -0,019 +0 | -0,03 +0 | -0,046 +0 | -0,074 +0 | -0,12 +0 | -0,19 +0 | -0,06 -0,134 | -0,03 -0,06 | -0,03 -0,076 | -0,03 -0,104 | +0 -0,046 | +0 -0,074 | +0 -0,12 | +0 -0,19 |
80 < D ≤ 120 | -0,022 +0 | -0,035 +0 | -0,054 +0 | -0,087 +0 | -0,14 +0 | -0,22 +0 | -0,072 -0,159 | -0,036 -0,071 | -0,036 -0,09 | -0,036 -0,123 | +0 -0,054 | +0 -0,087 | +0 -0,14 | +0 -0,22 |
120 < D ≤ 180 | -0,025 +0 | -0,04 +0 | -0,063 +0 | -0,1 +0 | -0,16 +0 | -0,25 +0 | -0,085 -0,185 | -0,043 -0,083 | -0,043 -0,106 | -0,043 -0,143 | +0 -0,063 | +0 -0,1 | +0 -0,16 | +0 -0,25 |
180 < D ≤ 250 | +0,029 -0 | +0,046 -0 | +0,072 -0 | +0,115 -0 | +0,185 -0 | +0,29 -0 | -0,1 -0,215 | -0,05 -0,096 | -0,05 -0,122 | -0,05 -0,165 | +0 -0,072 | +0 -0,115 | +0 -0,185 | +0 -0,29 |
250 < D ≤ 315 | +0,032 -0 | +0,052 -0 | +0,081 -0 | +0,13 -0 | +0,21 -0 | +0,32 -0 | -0,11 -0,24 | -0,056 -0,108 | -0,056 -0,137 | -0,056 -0,185 | +0 -0,081 | +0 -0,13 | +0 -0,21 | +0 -0,32 |
315 < D ≤ 400 | +0,036 -0 | +0,057 -0 | +0,089 -0 | +0,14 -0 | +0,23 -0 | +0,36 -0 | -0,125 -0,265 | -0,062 -0,119 | -0,062 -0,151 | -0,062 -0,202 | +0 -0,089 | +0 -0,14 | +0 -0,23 | +0 -0,36 |
400 < D ≤ 500 | +0,04 -0 | +0,063 -0 | +0,097 -0 | +0,155 -0 | +0,25 -0 | +0,4 -0 | -0,135 -0,29 | -0,068 -0,131 | -0,068 -0,165 | -0,068 -0,223 | +0 -0,097 | +0 -0,155 | +0 -0,25 | +0 -0,4 |
Common Problems For Hydraulic Cylinders
7.0. COMMON PROBLEMS FOR HYDRAULIC CYLINDERS
7.1. AIR IN OIL
Air in oil problem is found mostly in mobile hydraulics. These include three important effects such as jet cut effect, diesel effect and cavitation.
7.1.1. JET CUT EFFECT
Air can be soluble or insoluble in the media. There is molecular soluble air in all the hydraulic oils. Gas molecules are possibly mixed or bond to oil molecules. Depending on type of media, the rate of soluble air will vary. Oil pressure capability, viscosity and workability characteristics will not to be affected by soluble air.
Insoluble air will cause the oil to behave erratically especially at low pressures (approx 60 bar). For example, as media speed increases, air bubbles will be dispersed over varying distances.
If the pressurised media contains insoluble air, this air is pressurised and will find its way to the seal groove. Subsequently, these air bubbles will be freed when the pressure decreases which lead to the emission of tremendous energy brought about through air expansion. As a result, metal surfaces are affected and surface roughness is increased.
As a result of these explosions if the cracks on the sealing element are on the direction of the cylinder, these small channels cause a nozzle effect. As the speed of oil increases, it makes a jet effect on these nozzles and causes cuts on these parts. Meanwhile, oil molecules pass the seal at a high speed, penetrating behind and wearing the back of it. If there is too much insoluble air in the oil, this expansion might tear the seal out half. This type of damage will commonly occur with rubber fabric products which have been immersed in rubber solution. The reason being, these products contain too much porosity and air transferability other then homogenised rubber seals.
This type of damage can be stopped with increasing the dimensions of the groove at the design stage. The real reason for this damage is escaped pressurised air penetrating behind the seal rather than extrusion. Pressured air bubbles damage
the sealing element when they expand by being absorbed also by homogenised elastomer sealing elements. If these seals are removed for inspection, damage can be found on seals hydraulic sealing lips. The seal volume is expanded and material becomes softer.
Pressure shocks can be with short strokes in the hydraulic systems. Air bubbles in the system are loaded with high temperature energy. At the ideal gas formulation, pressure is to be a positive function of temperature and temperature will increase with pressure increase at the same time. If heated air bubbles are expanded, they start to wear the sealing element and tear pieces from it with high temperature and force. In this area, researches show that the temperature of these air bubbles could be far more than 200°C, even reach to 1000°C. This temperature changes depending on the size of the air bubbles before pressure, pressure, speed and load.
7.1.2. DIESEL EFFECT
Most serious damages in hydraulic cylinders originate from diesel effect explosions of the air in the oil. Speedily pressured air quickly reaches so high temperatures that it causes air and oil mixture to explode with fire. This situation is seen more often in cylinders that work against unsteady loads. While this explosion, pressure will cause an increase 5-6 times more then nominal working pressure. Consequently sealing, guidening elements and metal surfaces are damaged. This damage is observed as local burn and melting on sealing elements and thermoplastic parts.
Because of this reason some extra applications are needed to prevent air in oil in oil tanks, pumps valves and cylinders. Maintenance engineers should be sure about there is not any air left in the system on replacing the cylinder. Otherwise jet and diesel effects would damage the sealing element.
Hydraulic system is on danger when soluble air limit is exceeded on normal pressure. Even sometimes under limit saturation related vacuum might create air from system oil with evaporation and seal can be damaged (pls. see Cavitation part). If any problematic cylinder seals inspection is needed, inspection should be carried out with the seal designer and manufacturer at the same time while removing the seal from cylinder. Because replacing the sealing element would not solve the problem.
7.1.3. CAVITATION
Pressured hydraulic media speed increases while transferring through a rod (for example valve). On Bernoulli Formula (Pst+Pdyn=constant),when speed related dynamic pressure increases static pressure drops and it can be continued to create vacuum. After that as a result, soluble air in the oil is evaporated like steam drops. This is called as Cavitation.
These steam drops explode while transferring to pressure point. If this explosion occurs on a sealing element or a metal surface, the force created by the explosion damages the surfaces. This is called jet erosion.
There is small possibility of cavitation in systems working with hydraulic oil, because steam pressure of oil is too low (1.5 - 2.5 Torr.) However, there is a possibility of cavitation with water processed systems, because water steam pressure is 0.3 BAR and the created force is enough to damage even metal surfaces.
RESULT
Insoluble air in oil is very dangerous for hydraulic systems. How does the air come into being and how can we prevent it?
- System start-up, assembly or de-assembly can create air. Air has to be removed during a new pump, valve or piston assembly or when repair work is carried out for maintenance. For example, prior to the pumps starting a competent person should take the air out from air valves by turning the rod mil or cam by hand. In the same way, air should be taken out of pistons and hose or hose fittings have to be properly connected.
- Loose fitting elements can cause air transfer to system. Using poor quality fitting materials is a very common cause. And on the other hand, fitting elements frequently become loosened while they are working under shock and vibrations (like heavy duty machines). Because of this reason they should be subject to periodic checking and if possible, chemical compounds that increase the sealing should be used.
- Sometimes machine design factors cause air transfer to systems. The majority of machine designers work on minimal tank volume and pump positioning because of space limitations. Oil tank volume should be within the safety limit when oil is used by all the system and whilst the pump is operational. Moreover, the oil return outlet should not be positioned too close to the oil pump inlet or too high and create oil turbulence. Heavy duty seals should be used especially in the manufacturing of heavy machine pistons which work under shock and vibrations. Sealing element extrusion gap (groove) should be bigger and seal supported with guidening rings.
7.2. HYDRODYNAMIC PRESSURE PROBLEM
Hydrodynamic pressure is a common problem of hydraulic cylinders. The shortest definition of Hydrodynamic pressure is that the pressure in the space between the sealing element in hydraulic cylinders and the guidening element causes a type of permanent seal deformation by reaching a value far beyond the system pressure. Prior to the explanation, there is a list of key terminology provided for your convenience.
AB: inlet-outlet ports for hydraulic cylinder
C: Piston head
D: Cylinder cap
E: Rod
F: Guidening element
G: Rod seal
H: Piston Seal
I: Wiper
S: Gap between rod and guidening element
In double acting hydraulic cylinder drawing as seen in figure 10, pressured media enters from A port and leads the movement of the rod to the right. At the same time pressured media fills the gap between rod and guidening element “S” and the gap in front of the rod seal. If pressured media is sent to B side and A side is made tank, rod starts to move left along the cylinder stroke length with pressure effect.
Meanwhile if tight tolerances have been applied in “S” gap, it is evident that much of the hydraulic media which remains on the front surface of the seal can not make a tank from the A line. In this application, at every stage the rate of media oil increases and the system starts to work like a pump and creates more pressure then normal. Consequently, the seal and possibly cylinder are damaged.
In figure 11 the rod section in the hydraulic cylinder design has been removed and the chart shows the increase of hydrodynamic pressure (ph). The result of this increase with repeated movement can be calculated with the empirical formula below.
Ph= 6V.LY.1/S2
As can be seen in the equation above, hydrodynamic pressure sliding speed is directly related to guidening length, dynamic viscosity of the media and gap between rod and guidening element.
V= Sliding speed (m/sn)
ly= Guidening length (m)
l= Dynamic viscosity (Pa.sn)
S= Gap between rod and guidening (mm)
Some hydrodynamic pressure advice and solutions are given in the paragraphs below. Reducing the three figures which are given in the numerator of the hydrodynamic formula (sliding speed, guidening length and dynamic viscosity) may seem like the solution but generally speaking, due to possible hydraulic design problems, we should not touch these figures. If the rate of the gap between the guidening element and the rod is increased, it can be seen that there will be a decrease in hydrodynamic pressure value proportional to the square root of that increase. As a result of “S” gap being widened, guidening element in the hydraulic cylinder will not function properly
and there will be more serious problems with the effect of perpendicular forces in the hydraulic cylinder.
Some of the parts that are used as guidening elements in hydraulic cylinders are as follows;
- Phenolic resin (fibres) guides
2. Castled guides
3. Bronze guides
4. Polyacetal (POM) or polyamide (PA) guides
5. PTFE Guides
6. Special Teflon coated metallic rings.
Depending on the type of guidening element, it is evident that the solutions may vary. By opening helical grooves on cast iron or bronze guidening elements that are used in Figure 12, media accumulation that may occur on the front of sealing element can be foiled.
If it is not possible to create helical grooves, as seen in Figure 13, hydrodynamic pressure can be prevented by making a drain hole in rod seal housing.
If tape guides are used, as seen in Figure 14, the most important factor is that there must be a ‘k’ gap on the guidening elements. A gap should be left as shown in figure represented as ‘k’. Hydraulic media which remains on the front of the seal during the process should be returned to the system from this gap.
The minimum recommended ‘k’ gap values are as follows;
Ø |
k |
20-40 |
1 |
40-70 |
1.5-2 |
70-100 |
2-2.5 |
100-200 |
2.5-3.5 |
200-250 |
3.5-4 |
CONCLUSION
Hydrodynamic pressure can cause damage to hydraulic sealing elements and the hydraulic cylinder parts. Therefore, the build up of hydrodynamic pressure should not be permitted in hydraulic cylinder design and applications.
Surface Roughness
6.0. SURFACE ROUGHNESS
Surface roughness is an important parameter for sealing elements to work at desired effectiveness. Especially the lip part of the dynamic sealing elements are designed to provide sealing by sliding on an oil film thickness. This oil film thickness affects the sliding speed and life of the sealing element in a positive way. A high roughness of the sliding surface causes the exposure of the sealing element to dry friction by tearing the oil film thickness. This is an undesirable situation. Particularly the dynamic surfaces that touch the sealing element should be honned and grinded in order to obtain desired roughness value.
Surface roughness is measured by a device called profilemeter in terms of (μm). The measurement and calculation of the surface roughness is shown below.
Ra=Average Roughness Value
Arithmetic average of absolute values.
Rmax=Maximum Roughness Value
It is the maximum surface roughness value on the measuring line
Rz=Roughness Value of which average is taken
It shows the arithmetic mean of surface roughness depth separately (from Z1 to Z5) of five consecutive and different groups.
Rz= (Z1+Z2+Z3+Z4+Z5)/5
Depending on their production method, surface roughness should be between the values indicated in the table below.
Tolerance: It is the difference between the highest and the lowest measures that can be allowed. One of the important factors that affect the working of the sealing element at desired effectiveness is the tolerances of the seal housing, rod and tube. Unsuitable tolerances may cause serious problems in the system as well as affect the life cycle of the sealing element.
ISO tolerance system is used in hydraulic and pneumatic cylinders.
Design Criteria in Hydraulic Cylinders
5.0. DESIGN CRITERIA IN HYDRAULIC CYLINDERS
There are so many factors involved in sealing elements working principle. Ignoring any of these factors could increase the possibility of a design mistake. Hydraulic cylinders have different working conditions. For example, the cylinder used in injection machine and loader both have different seal design criteria in terms of material and sealing elements.
Four main variables should be taken into account while choosing a sealing element.
1. Temperature:
Media and working condition temperature should be considered when choosing suitable sealing element.
2. Pressure:
System pressure and pressure type should be considered when choosing suitable sealing element.
3. Sliding Speed:
System sliding speed should be considered when choosing suitable sealing element.
4. Media:
Media type should be considered when choosing suitable sealing element.
The technical values related to these 4 main variables can be found in our technical catalogue for each sealing element. Apart from these 4 main criteria, there are other variables which also affect the working of hydraulic cylinders. Alp Seals consider these following conditions to be the most important ones.
5. Working Conditions
This is one of the most important variables for hydraulic cylinder design. There are so many factors on this stage which deserve attention.
Main factors are as follows;
5.1. Working in an open or closed area and dirty working conditions are most important criteria for choosing a wiper. It may be necessary to use an ice scraper in winter for cylinders in outside working conditions.
5.2. Radial loads and working positions that affect hydraulic cylinders should be taken into consideration when deciding on the hydraulic cylinder design. For example; rain drops should be prevented from entering into the vertical functioning hydraulic cylinders that are in outside working conditions.
Sealing Element Drawing and Measurement Techniques
4.0. SEALING ELEMENT DRAWING AND MEASUREMENT TECHNIQUES
Because the sealing elements are produced generally from elastomer with a moulding technique, measurement technique is different from that of mechanical engineering. For example, only the inner and outer diameters of a circular product are commonly measured in mechanical engineering.
This type of measurement is suitable for machining technology. However, it is not possible to take measurements with ordinary measurement tools (like callipers, micrometers) on seal products because of their elastic form.
Measurements which are illustrated in technical drawings below will afterwards enable easier product measurement. In this technical drawing, a diameter length has been provided. The dimensional tolerance is bigger compared to steel products.
Because of this reason, it would not be wrong to think that measurement mistakes will stay within tolerance. We can see that the all other dimensional measures of the sealing element are on the cross section.
Measurement would be made easily with a sample cross section from the sealing element and with the help of a measurement device like a profile projector or microscope.
In the table below, permissible tolerances for elastomer products (ISO 3302-1 1999 edition) can be seen. Here in this table, Class M2-F column is used which is used by world class sealing element manufacturers. It should be kept in mind that these tolerances demonstrate the maximum figures. Another important point is that and it is not always necessary to use ± deviation values as stated in that table. Seal designers always take into account working conditions of the seal and then decide on + or – tolerance staying within the permissible dimensions.
TABLE-3-TOLERANCES FOR ELASTOMER |
|||||||||
|
CLASS M1 |
CLASS M2 |
CLASS M3 |
CLASS M4 |
|||||
NOMINAL DIMENSION |
F |
C |
F |
C |
F |
C |
F |
C |
|
Permissible deviations of dimensions in mm |
|||||||||
≤ 6,3 |
± 0,10 |
± 0,10 |
± 0,15 |
± 0,20 |
± 0,25 |
± 0,40 |
± 0,50 |
± 0,50 |
|
6,3< |
≤10 |
± 0,10 |
± 0,15 |
± 0,20 |
± 0,20 |
± 0,30 |
± 0,50 |
± 0,70 |
± 0,70 |
10 < |
≤16 |
± 0,15 |
± 0,20 |
± 0,20 |
± 0,25 |
± 0,40 |
± 0,60 |
± 0,80 |
± 0,80 |
16< |
≤25 |
± 0,20 |
± 0,20 |
± 0,25 |
± 0,35 |
± 0,50 |
± 0,80 |
± 1,00 |
± 1,00 |
25< |
≤40 |
± 0,20 |
± 0,25 |
± 0,35 |
± 0,40 |
± 0,60 |
± 1,00 |
± 1,30 |
± 1,30 |
40< |
≤ 63 |
± 0,25 |
± 0,35 |
± 0,40 |
± 0,50 |
± 0,80 |
± 1,30 |
± 1,60 |
± 1,60 |
63< |
≤100 |
± 0,35 |
± 0,40 |
± 0,50 |
± 0,70 |
± 1,00 |
± 1,60 |
± 2,00 |
± 2,00 |
100< |
≤160 |
± 0,40 |
± 0,50 |
± 0,70 |
± 0,80 |
± 1,30 |
± 2,00 |
± 2,50 |
± 2,50 |
Permissible deviations in (%) |
|||||||||
160< |
|
0,30 |
|
0,50 |
|
0,80 |
|
1,50 |
1,50 |
Extract from ISO 3302-1 (1999 EDITION) |
TABLE 4-TOLERANCES FOR MACHINED PARTS IN PTFE OR OTHER THERMOPLASTICS |
|||
NOMINAL DIMENSION |
TOLERANCE ACCORDING TO DIN 7168 ‘’MEDIUM’’ |
RESTRICTED WORKS TOLERANCES |
|
≤ 6 |
± 0,1 |
0,10 |
|
6< ≤ 30 |
± 0,2 |
0,15 |
|
30< ≤ 65 |
± 0,3 |
0,20 |
|
65 < ≤ 120 |
± 0,3 |
0,30 |
|
120 < ≤ 200 |
± 0,5 |
0,40 |
|
Extract from DIN 7168 |
Sealing Mechanism
3.0. SEALING MECHANISM
If we want to create a pressure difference between two different areas in hydraulic systems, the necessity of sealing arises.
As seen in diagram-1, depending on the required pressure difference, a leakage occurs within the gap between rod and cylinder.
Where, pressure difference = ΔP
If ΔP =P1-P2
Q, the oil flow rate from ‘S’ gap, will be
Q = π.d. ΔP.S3 / 12.μ.L
Where, Q= Flow rate μ=Viscosity
In real applications, the “S” gap between both sides of the piston rod is not always equal. Due to radial forces there may be excessive contact on one side. This means that the the “S” gap will increase on one side.
If we look at the formula above, the “S” gap shows a twofold increase which is proportional to the cubed volume therefore resulting in an eightfold increase in passing oil rate. In practice, it is not possible to set the production tolerances of the “S” gap at zero.
Therefore, if a leakage is not desired, we are obligated to use a sealing element in this section.
Seals made of elastomer materials are generally used in this kind of applications. When analysing the way in which seals work, we can see in figure-2 the logic behind the role of the O-ring. The O-ring illustrated in the diagram is depicted as being dynamic and functions as a rod sealing element. According to the general principle, after being fitted into the groove, a sufficient amount of space must remain in the groove.
When the system is in unpressurised condition, assembled O-ring, because of its design, applies a pressure on the Rod. We call this a pre-load. Pre-load is essential to provide sealing in unpressurised conditions.
When the system is pressurised, there must be a construction which allows the pressured media through as far as the sealing element housing. This pressured media which starts to fill the sealing element groove, leads to an increase in pressure force applied on static and dynamic surfaces by expanding the seal material. In this way, sealing is obtained.
Below in figure-3 the result of fea (finite element analysis) can be seen. In this analysis, the working of an O-ring has been simulated digitally. The different colours used enable us to see clearly material deformation under critical loads.
We should not ignore the fact that this critical loads can increase and sealing materials can be viscosed i.e. there can be leakage problem because of the space on one side in the unpressurised part of the system. Therefore, seal manufacturers should show particular care when designing seals and hydraulic piston manufacturers should pay more attention in choosing the right seal product to improve the quality of their end product.
If there is not enough space between seal and groove, pressured media can’t expand the sealing element material which will cause excess friction forces and the seal will temporarily be deformed thus creating a leak.
3.1. STATIC SEALING
The increase in the contact pressure by the sealing element will reduce oil leaks in static sealing. Contact pressure in its optimum level will stop oil leaks completely. Of course in the meantime, an important factor which must not be ignored is the roughness of the application surfaces. Naturally, high roughness values require higher contact pressure.
Sealing materials will give different elastic and compression set results under different working temperatures. Therefore, Temperature differences may create really important functional differences. Low temperatures, in particular, may cause sealing materials to lose elasticity and become hard, resulting in the seal failing to work.
3.2. DYNAMIC SEALING
While we inspect dynamic sealing, we should look at the working principle which is explained by the flow formula;
Q = π.d. ΔP.S3 / 12.μ.L
In this formula, the rod is static and pressure is decreasing. If the rod is dynamic and pressure is not decreasing.
Q1 = π.d.S.V/2
Flow rate can be calculated with V= speed formula. If the piston is moving with the pressure direction, Q dynamic = Q+Q1
That is to say,
Q dynamic = π.d ( ΔP.S3/12. μ.L ± V.S/2)
In this formulation speed and direction of the speed will be of great importantance. On the other hand, if S gap is not parallel because of radial forces, extra pressure will be created locally (hydrodynamic pressure).
Because of this effect in microscopic size, force will be created in the hydrodynamic oil film between seal and dynamic surface. This oil film is only a few micron thick.
This oil film thickness is proportional to direction of the movement. Consequently, it is not possible to have leak free systems on dynamic applications. Nevertheless, oil film thickness can be decreased or increased depending on application type and seal geometry.
3.3. FRICTION FORCE
Friction force is not possible to be explained with classic friction force laws because of a slide on oil film thickness as explained above. 3 different friction forces occur because of the oil film:
1. Static (dry friction)
2. Mixed friction (dry and media friction)
3. Media friction (there is not any dry contact)
When the dynamic movement starts, a high friction force arises which leads to a mixed friction, resulting in the immediate decrease in friction force. After this, media friction i.e. a slide on oil film thickness begins. As the speed increases, so the friction force increases linearly.
The oil film thickness is related to the system speed and directly to media viscosity. Two most important factors that affect the friction force are system pressure and temperature. Unsuitable friction forces create local high temperature and will result in seal failure and breakdown.
At the same time wear arises because of the tears in oil film thickness and with the effect of unsuitable surface roughness values, which results in leakage.
Commonly Used Medias For Hydraulic Systems
2.0. COMMONLY USED MEDIAS (FLUIDS) FOR HYDRAULIC SYSTEMS
These oils, which comply with the DIN 51524 standards, are commonly used in hydraulic systems. There are 5 different categories.
DIN 51524 MİNERAL OILS |
||||
MEDIA |
STANDARD |
SPECIFICATIONS |
APPLICATION AREA |
INTERNATIONAL TERMİNOLOGY |
H-L Type Oils |
DIN-E 51524 part 1 |
Contains oxidisation retardant and rustproofing agent. |
General Usage |
HL |
H-LP Type Oils |
DIN-E 51524 part 2 |
H-L Type oils are added to prevent abrasion. |
High Pressure Systems |
HM |
H-V Type Oils |
DIN-E 51524 part 3 |
H-LP Type oils are added as a heat regulating agent. |
Various Temperature Systems |
HV |
H-LPD Type Oils |
|
H-LP Type oils contain only a limited amount of detergent and water. |
Processes in which water is used |
|
H-D Motor Oils |
API-CC |
Oxidisation and rustproofing agent which reduces abrasion. |
Mobile Hydraulic Systems |
|
However, it may not always be possible to use mineral oils in hydraulic systems. It is important to consider the type of media being used when choosing the appropriate seals.
The table below shows commonly used media types and the areas in which they are used.
MATERIAL |
Imflammable Hydraulic oils - VDMA 24317 or DIN 24320 standards |
|||
HFA |
HFB |
HFC |
HFD |
|
70 NBR |
55 |
60 |
60 |
|
80 NBR |
55 |
60 |
60 |
|
90 NBR |
55 |
60 |
60 |
|
70 FKM |
55 |
60 |
60 |
150 |
80 FKM |
55 |
60 |
60 |
150 |
90 FKM |
55 |
60 |
60 |
150 |
80 PU |
40 |
40 |
40 |
|
90 PU |
40 |
40 |
40 |
|
PTFE |
|
|
|
|
TPE |
40 |
40 |
40 |
|
POM |
55 |
60 |
60 |
80 |
Sealing Element Materials
1.0. MATERIALS (COMPOUNDS) USED FOR HYDRAULIC AND PNEUMATIC SEAL PRODUCTION
1.1. Plastomers (Thermoplastics)
These materials with macro molecular structure are called thermoplastics or engineering plastics.
On examination of the molecular architecture, it can be seen that, unlike elastomers, there are no cross links present. Although this product is resilient under system pressure, that is to say it is able to return to its original form, its performance is lower compared to elastomers.
Polyacetal, polyamide and PTFE (Teflon) materials are generally used plastomers in sealing elements.
1.1.1. Polyacetal (POM) (Polyoxymethylene)
These are used as back-up rings or guidening element materials in hydraulic and pneumatic systems.
There are two types of POM, filled with or without fibreglass, depending on their use. Despite working temperatures ranging from -40°C to +140°C, real dimensions can not remain
stable in excess of 100°C.
It can be used safely with mineral oils and HFA, HFB type oils. Types with added fibreglass provide longer lasting contact pressure resistance.
1.1.2. Polyamide (PA6, PA6, 6)
This material can be used at peak temperature of 140°C and can retain its dimensions between -40°C and 100°C.
Like Polyacetals, Polyamids can be used as back-up rings and guidening elements in Hydraulic-Pneumatic cylinders. They are available with or without fibreglass.
1.1.3. PTFE (Teflon)
In 1938, in the DUPONT Laboratories, Dr. Roy J. Plunket Freon happened upon a white waxy substance whilst carrying out some tests. On further analysis, the culmination of the research revealed that the substance contained important qualities for use in industry.
In production technology, PTFE, whose production technique resembles that of powder metallurgy, in its powdered form is moulded as rods or tube and sintered in owen. However, as
a result of technological progress over the last 15-20 years, injection and extrusion methods are used on materials such as thermoplastics, which have become less popular, and therefore less widely used.
Teflon can be used as virgin, or used within fillers in order to improve mechanical performance. The fillers’ effects on PTFE are to improve extrusion resistance, reduce friction and form changes under extreme heat conditions. In addition, it increases resilience to attrition and hardening.
The most important fillers are;
-Fibreglass,
-Carbon-graphite,
-Colouring pigments,
-Bronze and molybdenum disulfide.
1.1.3.1. Fibreglass
Fibreglass filler provides chemical resistance in a wide range of working temperatures. It is especially resistant to powerful alkaline solutions and hydrofluoric acids.
1.1.3.2. Carbon-Graphite
It improves resistance to wear and hardness. Teflon filled with Carbon-Graphite prevents quick wear on tool inserts during machining and provides high pressure resistance.
1.2.5. Neoprene (CR)
CR is a cholorobutadiene polymer. It is resistant to air, ozone and flame. It has good resistance to mineral oils with high aniline points and greases. Thus, it is used in applications which need to be resistant both to oils and air and ozone at the same time.
For example; rod and rod end bellows in automotive sector are produced from neoprene materials. Working Temperature Range: -45°C to +105°C.
1.2.6. Ethylene Propylene Rubber (EPDM)
It has excellent resistance to phosphate, ester fluids, automotive break fluids, water and steam. Working Temperature Range: -40°C to +145°C
1.2.7. Styrene Butadiene Rubber (SBR)
It has good resistance to glycol based automotive break oils, inorganic acids, base chemicals and alcohol. Often used with other elastomers e.g. natural rubber. Working Temperature Range: -50°C to +100°C
1.2.8. Polyurethane (PU)
PU is moulded with injection techniques. It has excellent mechanical properties such as high tensile strength, wear resistance, tear strength, and extrusion resistance. It offers a good resistance to air, ozone, mineral oils, grease and aliphatic hydro carbons. Working Temperature Range: -30°C to 100°C.
1.2.9. Natural Rubber (NR)
NR is produced from rubber tree. It can be used when high mechanical resistance and elasticity is necessary (for example, vibration absorber blocks etc.). Working Temperature Range: -60°C to 100°C.
1.3. THERMOPLASTIC ELASTOMERS (TPE)
TPE materials are widely used in hydraulic-pneumatic applications with a variety of combinations due to their high resistance to mineral oils and high tensile strength. Working temperature is between -40°C and +120°C
1.1.3.3. Molybdenum di Sulphite
Improves hardness and reduces friction force. It is used with the other fillers.
1.1.3.4. Bronze
Improves thermal resistance, high pressure stability and extrusion resistance. It is the most preferred filler for hydraulic applications.
1.2. ELASTOMERS
These differ from plastomers in the respect that the macro molecular structure comprises of cross links. Due to this characteristic, these are highly superior visco-elastic products. These products need to contain the properties of both liquidity and elasticity. Elastomers are derived from the sap of the rubber tree, which is extracted by penetrating the bark, and is collected and modified with sulphur.
Materials which can be stretched to twice their original size before returning to their original dimensions at room temperature are called polymeric elastomer products.
Macro molecular cross links can be produced with the effect of heat and pressure. This process is called vulcanization (cross link reaction) and gives the material its perfect elastic properties.
The most commonly used elastomers are explained below;
1.2.1. Nitrile (NBR)
Nitrile is a widely used nitrile butadiene and acrylo nitrile polymer.
Alp Seals NBR formulas contain between 30% and 50% acrylo nitrile (ACN). Our NBR which has a working temperature between -30°C and +105°C (peak temp. at +130°C) show extremely high resistance under different working conditions such as mineral oils (H, H-L and H-LP type lubricating oils) and grease and mineral based fire resistance oils (HFB and HFC group oils), vegetable and organic oils, and aliphatic hydrocarbons (propane, butane, petrol )
Strongly not recommended for aromatic hydrocarbons (benzene, trichloroethylene, HFD type fire resistance oils), glycol and ether contained break hydraulics.
1.2.2. Hydrogenated Nitrile Butadiene Rubber (HNBR)
HNBR rubber, is a fully or partial hydrogenated standard NBR polymer with double linked butadiene. Its chemical resistance is similar to NBR. If it is vulcanized with peroxides, working temperature and mechanical durability can be extended. Working Temperature Range is between -30°C to +130°C.
1.2.3. Viton (FKM) (FLUOREL ELASTOMER)
It is resistant to any type of grease oil and solvent between -30°C and +225°C. Better results can be obtained in low gas transfer systems. It has a good resistance to a range of different chemicals (especially greases, fuels, aliphatic and aromatic hydrocarbons, some fire resistant oils).
1.2.4. Silicone (MVQ)
Silicone which is highly resistant to ozone, air and oil, protects its characteristics between -60°C and +200°C. Yet, it has poor resistance to oxidised oils, some of hypoid and E.P type oils).
APR HEAVY DUTY POLYESTER GUIDE STRIP
APR HEAVY DUTY POLYESTER GUIDE STRIP
- ULTRA LOW FRICTION
- VERY ECONOMIC BEARING SOLUTION
- EXCELLENT STABILITY UNDER OPERATING TEMPERATURES
- RESISTANCE TO HEAVY LOADS UPTO 100 NEWTON/MM2
- STICK SLEEP FREE
- SUITABLE TO WORK UNDER DRY CONDITIONS
- LONG SERVICE LIFE
- USED FOR HEAVY DUTY HYDRAULIC AND PNEUMATIC CYLINDERS